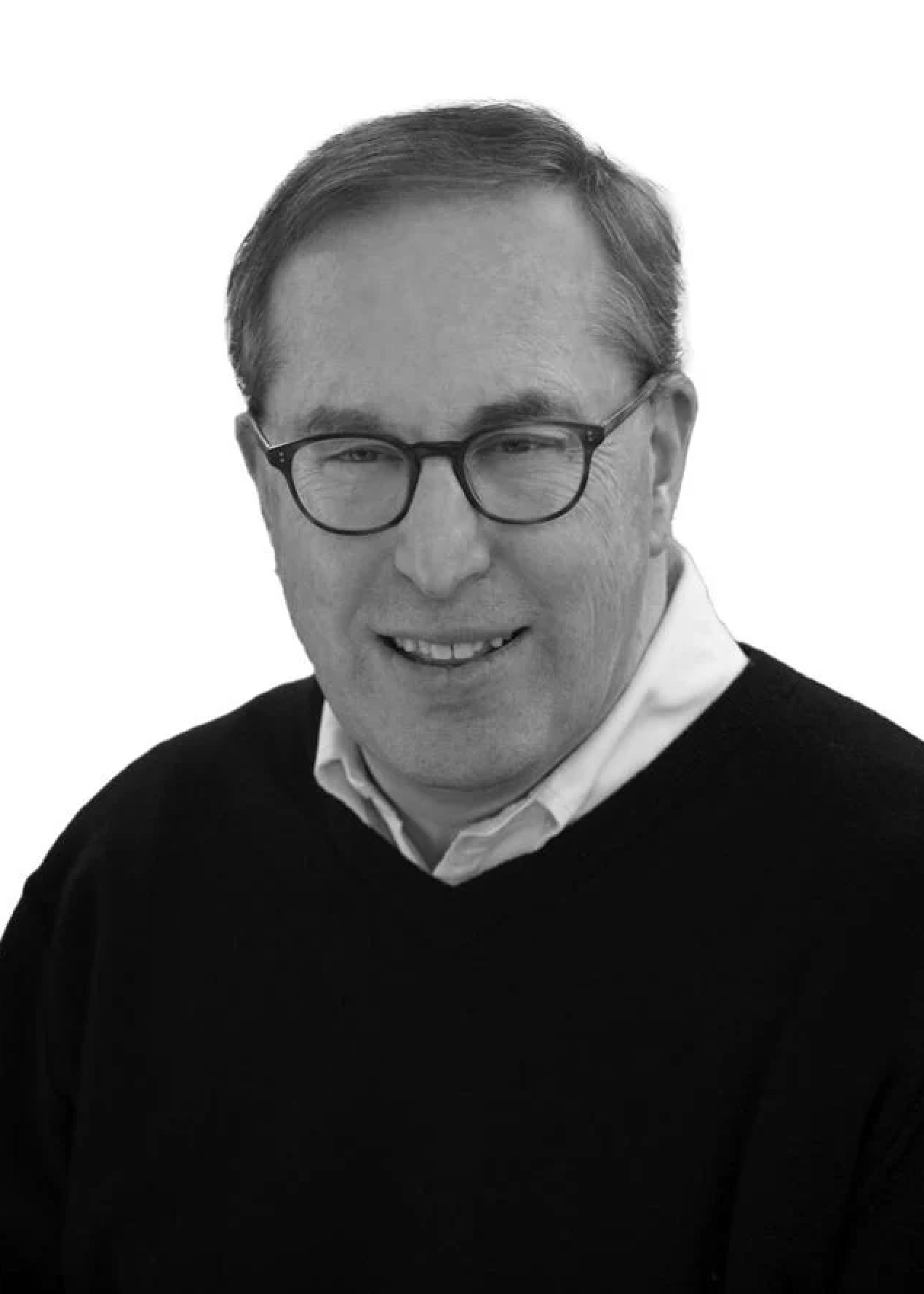
Quantum mechanics — the breakthrough physics theory that paved the way to our modern understanding of atoms, molecules and light — is turning 100 years old next year.
During that time, the theory has generated many technological breakthroughs, such as nuclear energy, the laser and semiconductor chips; yet the fundamental conceptual framework hasn’t changed. So, why have America’s most illustrious tech giants (Google, Microsoft, IBM, Amazon), as well as national funding agencies around the globe, recently decided to pour millions and even billions of dollars into new research centers, all designated with the letter Q?
The reason: a re-examination of the theory that revealed unsuspected properties of great interest and potential utility, hiding in plain sight.
The “Second Quantum Revolution” began when physicists, mathematicians and computer scientists started thinking about what quantum mechanics implies for the storage, processing and transmission of information, creating a potentially transformative field: quantum information science. In a historic irony, the field hinges crucially on the weird features of quantum mechanics that physicists tended to ignore, but which have seeped into the popular consciousness with buzzwords such as “Schrodinger’s Cat.” In this new field, the weirdness is the point!
The most striking idea of this new field is to store information as a “quantum object” (most simply, either an atom, or a nano-engineered “artificial atom”). Quantum objects are defined via three key concepts: quantization, superposition and entanglement. Quantization refers to the fact that a quantum object, such as a molecule, cannot exist with an arbitrary amount of energy. For example, when a molecule vibrates along a chemical bond, we can think of the bond as an elastic band. But according to quantum mechanics, this band behaves strangely, in that it can only be stretched by 1, 2 or 3 inches and nothing in between. This means the chemical bond can only store certain allowed amounts of energy, called “states,” unlike a macro elastic material. Thus, at the level of atoms and molecules, nature has some intrinsic graininess, too small to be seen in our macroscopic world.
It gets weirder. Before it is measured, a molecule can exist, simultaneously, in two (or more) such states called a superposition. When measured, each such molecule would randomly reveal one of the two quantized energy values, and never anything in between. However, which result you get on a single try is unpredictable. Importantly, according to quantum theory, the molecule, prior to measurement, is not in an unknown but a definite state; it is really in two incompatible states at the same time. Very weird.
Finally, there is entanglement. Imagine you prepare two molecules, each in a fuzzy superposition, so that the total energy of the two is fixed. Then, when you measured the energy of one of them, the energy of the other one would be determined, and no longer fuzzy, even if you had separated the two molecules by a large distance. Such a state of two molecules is called “entangled,” and a measurement of one appears to create a “spooky” connection to the other. Einstein felt that this must be wrong. However, starting in 1970 and up to the present, more and better experiments measured this entanglement property in excellent agreement with the predictions of quantum mechanics, a result recognized with the Nobel Prize in 2022.
All of these properties of quantum mechanics were known since the mid-1930s. But only in the 1980s did quantum scientists start to ask whether the weird aspects of quantum mechanics could be harnessed to do difficult and useful information-related tasks. It was proposed that binary information, a “one” or “zero,” could be stored in a quantum system with only two states, which could be defined as zero or one. This is now called quantum bit or qubit. But unlike regular digital bits, which are either definitely zero or definitely one, qubits can be stored in any of an infinite number of fuzzy, uncertain superpositions. This does not sound like progress.
However, remarkably, it was shown theoretically that a quantum computer that could store and process many such qubits would be able to solve problems that were beyond the power of any conceivable conventional computer, even if it ran for millennia. The most striking example is that an efficient quantum computer could break, in a reasonable time, one of the main codes used to securely transmit credit card information over the internet. However, to work better than a conventional computer, the quantum computer must create superposition and entanglement of qubits; the weirdness of quantum mechanics is a feature, not a bug!
This discovery initiated the race to build an efficient quantum computer, something more difficult than any previous project in nanoscience and engineering. If success is achieved, the benefits to society in areas like energy harvesting, environmental science and health science are potentially enormous.
Quantum mechanics — the breakthrough physics theory that paved the way to our modern understanding of atoms, molecules and light — is turning 100 years old next year.
During that time, the theory has generated many technological breakthroughs, such as nuclear energy, the laser and semiconductor chips; yet the fundamental conceptual framework hasn’t changed. So, why have America’s most illustrious tech giants (Google, Microsoft, IBM, Amazon), as well as national funding agencies around the globe, recently decided to pour millions and even billions of dollars into new research centers, all designated with the letter Q?
The reason: a re-examination of the theory that revealed unsuspected properties of great interest and potential utility, hiding in plain sight.
The “Second Quantum Revolution” began when physicists, mathematicians and computer scientists started thinking about what quantum mechanics implies for the storage, processing and transmission of information, creating a potentially transformative field: quantum information science. In a historic irony, the field hinges crucially on the weird features of quantum mechanics that physicists tended to ignore, but which have seeped into the popular consciousness with buzzwords such as “Schrodinger’s Cat.” In this new field, the weirdness is the point!
The most striking idea of this new field is to store information as a “quantum object” (most simply, either an atom, or a nano-engineered “artificial atom”). Quantum objects are defined via three key concepts: quantization, superposition and entanglement. Quantization refers to the fact that a quantum object, such as a molecule, cannot exist with an arbitrary amount of energy. For example, when a molecule vibrates along a chemical bond, we can think of the bond as an elastic band. But according to quantum mechanics, this band behaves strangely, in that it can only be stretched by 1, 2 or 3 inches and nothing in between. This means the chemical bond can only store certain allowed amounts of energy, called “states,” unlike a macro elastic material. Thus, at the level of atoms and molecules, nature has some intrinsic graininess, too small to be seen in our macroscopic world.
It gets weirder. Before it is measured, a molecule can exist, simultaneously, in two (or more) such states called a superposition. When measured, each such molecule would randomly reveal one of the two quantized energy values, and never anything in between. However, which result you get on a single try is unpredictable. Importantly, according to quantum theory, the molecule, prior to measurement, is not in an unknown but a definite state; it is really in two incompatible states at the same time. Very weird.
Finally, there is entanglement. Imagine you prepare two molecules, each in a fuzzy superposition, so that the total energy of the two is fixed. Then, when you measured the energy of one of them, the energy of the other one would be determined, and no longer fuzzy, even if you had separated the two molecules by a large distance. Such a state of two molecules is called “entangled,” and a measurement of one appears to create a “spooky” connection to the other. Einstein felt that this must be wrong. However, starting in 1970 and up to the present, more and better experiments measured this entanglement property in excellent agreement with the predictions of quantum mechanics, a result recognized with the Nobel Prize in 2022.
All of these properties of quantum mechanics were known since the mid-1930s. But only in the 1980s did quantum scientists start to ask whether the weird aspects of quantum mechanics could be harnessed to do difficult and useful information-related tasks. It was proposed that binary information, a “one” or “zero,” could be stored in a quantum system with only two states, which could be defined as zero or one. This is now called quantum bit or qubit. But unlike regular digital bits, which are either definitely zero or definitely one, qubits can be stored in any of an infinite number of fuzzy, uncertain superpositions. This does not sound like progress.
However, remarkably, it was shown theoretically that a quantum computer that could store and process many such qubits would be able to solve problems that were beyond the power of any conceivable conventional computer, even if it ran for millennia. The most striking example is that an efficient quantum computer could break, in a reasonable time, one of the main codes used to securely transmit credit card information over the internet. However, to work better than a conventional computer, the quantum computer must create superposition and entanglement of qubits; the weirdness of quantum mechanics is a feature, not a bug!
This discovery initiated the race to build an efficient quantum computer, something more difficult than any previous project in nanoscience and engineering. If success is achieved, the benefits to society in areas like energy harvesting, environmental science and health science are potentially enormous.
A. Douglas Stone is a professor in applied physics at Yale and deputy director of the Yale Quantum Institute, which has been a leading academic center for research in quantum computing. He has been a member, trustee and honorary member at the Aspen Center for Physics since 1994.