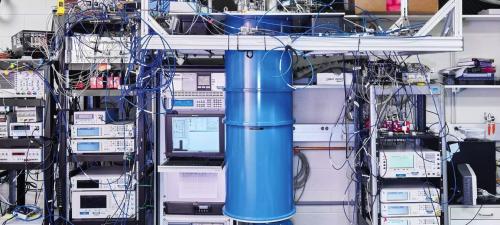
For decades, the promise of quantum computing has tickled the neurons of drug-makers, spies, and tech CEOs. Such a machine, if perfected, would speed up drug discoveries, decode ciphers, and help AIs parse our digital data. This new brain hinges on the bendy concept of superposition, the idea that an object can be in two states at once—a coin spinning so fast, it’s both heads and tails.
Conventional computer chips, whether in your phone or a supercomputer, hold transistors that process information as binary code: Everything is either a 0 or a 1. Quantum computers use qubits ( “cue-bits”), which can be both a 0 and a 1. The machines they inhabit can crack problems faster.
But there’s a rub: Qubits are fragile. Any interference can muddy computations. Yale University applied physicists Robert Schoelkopf and Michel Devoret pioneered a way to stabilize them. By building qubits out of superconductors—materials with no resistance to electrical current at extremely low temperatures—they create a space in which quantum algorithms can flow undisturbed. As a look inside their lab reveals, it takes a huge operation—and a very cold fridge—to help these tiny wafers think big.
For decades, the promise of quantum computing has tickled the neurons of drug-makers, spies, and tech CEOs. Such a machine, if perfected, would speed up drug discoveries, decode ciphers, and help AIs parse our digital data. This new brain hinges on the bendy concept of superposition, the idea that an object can be in two states at once—a coin spinning so fast, it’s both heads and tails.
Conventional computer chips, whether in your phone or a supercomputer, hold transistors that process information as binary code: Everything is either a 0 or a 1. Quantum computers use qubits ( “cue-bits”), which can be both a 0 and a 1. The machines they inhabit can crack problems faster.
But there’s a rub: Qubits are fragile. Any interference can muddy computations. Yale University applied physicists Robert Schoelkopf and Michel Devoret pioneered a way to stabilize them. By building qubits out of superconductors—materials with no resistance to electrical current at extremely low temperatures—they create a space in which quantum algorithms can flow undisturbed. As a look inside their lab reveals, it takes a huge operation—and a very cold fridge—to help these tiny wafers think big.
MR. FREEZE
By building qubits out of superconductors, they create a space in which quantum algorithms can flow undisturbed.
Spencer Lowell
↑ Qubits rely on many components. A wall of microwave generators create electromagnetic pulses that travel through a maze of coaxial cables and send the qubits—deep in the 5-foot-tall blue fridge pictured at the top of this article—into action. To create a climate colder than outer space, external pumps drive helium-3 refrigerant into copper tubing. As the helium circulates, it compresses, liquefies, and chills. It takes a day to hit the lowest low: 0.01 degrees Kelvin, or minus 459 degrees F.
FUNCTION JUNCTION
A nest of coaxial wires shuttle algorithms into and out of the fridge.
Spencer Lowell
↑ It takes graduate students, working in pairs, as long as two weeks to snake these wires through the giant cylinder to terminate here on the bottom of the chiller.
NOT A CHIP
The mighty qubit.
Spencer Lowell
↑ Behold the qubit, the cold and elegant heart of quantum computing. This 1-inch-long wafer is made of synthetic sapphire topped with a 100-nanometer-thin layer of printed aluminum. At insanely low temperatures, microwave photons send the junction point of the Y into super-position. The wavy strips below transmit the qubit’s results.
MEET-UP
Connector modules.
Spencer Lowell
↑ It takes groups of qubits to puzzle out anything more complex than a coin-flip. Modules like this one connect them. The Yale team designs these units for specific purposes. Some process data, some read it, others amplify it. Mastering this scalable, Lego-like approach could be more vital to perfecting quantum computers than piling on qubits.
SHHHH
Do not disturb.
Spencer Lowell
↑ It’s quiet inside the fridge. Any static can incite computing errors, so scientists must protect the qubits. A one-way valve, called a circulator (the four rectangles seen midframe in the photo above), filters out inter-ference, such as ambient lab noise.
GROUND CONTROL
The talent management.
Spencer Lowell
↑ Not all work happens in the cooler. A network analyzer (foreground) ensures that the microwave cabling needed to transmit signals is working properly. The tall gray cabinet directly behind it manages the refrigeration pumps and valves.
TEAR-DOWN
The building blocks of quantum computing.
Spencer Lowell
↑ Graduate students and postdocs are constantly building custom configurations to accomplish specific experiments. That can require piecing together dozens of qubits and qubit-nesting modules for new functions. Here, older modules (bottom left and middle right) sit among coaxial wires and signal filters.
TALL BOY
It takes a huge operation—and a very cold fridge—to help these tiny wafers think big.
Spencer Lowell
↑ Old quants work less. At 15 years old, this 9-foot-tall fridge is one of the lab’s elders. It doesn’t get the attention younger kids do. The narrow frame makes wiring it tricky, and chilling it requires lifting a huge cooling vat from a manhole in the floor. Still, if there’s no vacancy in the newer setups, students will pull this out for quick research.